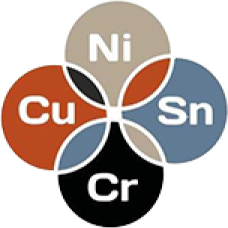
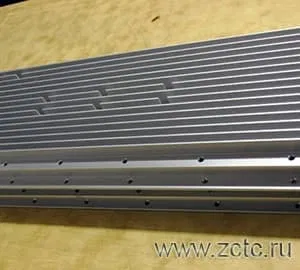
Mechanism and technology of anodizing An.ox. Structure and properties of aluminum oxide in the coating.
Содержание:
1. General information on anodizing (anodic oxidation) of aluminum
2. Composition and structure of aluminum oxide in the coating.
3. Theories of the formation of aluminum oxide films.
4. Особенности роста оксида алюминия при анодировании.
5. Features of the growth of aluminum oxide during anodizing.
6. Sealing and painting of anodic oxide films on aluminum.
7. Colored anodizing of aluminum
1. General information about anodic oxidation (anodization) of aluminum.
Due to the tendency to passivation, the surface of aluminum and its alloys is permanently covered with a natural oxide film. The film thickness depends on the ambient temperature and is usually 2-5 nm. The corrosion and mechanical strength of aluminum can be increased tens and hundreds of times by subjecting it to electrochemical oxidation (anodization).
Anodizing is the process of obtaining a protective oxide film from aluminum. Produced by electrochemical method in aqueous solutions. The density of such a coating is 2.9-3.8 g / cm3, depending on the production mode.
The purpose of the coating is to protect products from corrosion, increase wear resistance, and give a decorative appearance (when filled with dyes)
Designation |
Anodize; Anodic oxidation; An.Ox; An; Black-anodizing of metals with inorganic dyes |
Coating thickness |
6-40μm (for An.ox hardness, the thickness is higher) |
Microhardness (depends on the grade of aluminum alloy) |
1960-2450 MPa - D1, D16, V95. 2940-4900 MPa - A5, A7, A99, AD1, AMg2, AMg2s, AMg3, AMg5, AMg6, AMts. 4900 MPa - for enamel coating. |
Specific electrical resistance at 18 °C |
1012 Om⋅m |
Permissible operating temperature |
100° C (may increase when filled with dyes) |
Anodic oxide coatings are divided into the following groups:
- protective (9-40 microns) - requirements are imposed only on corrosion resistance;
- protective and decorative (9-40 microns) - not only corrosion resistance is important, but also appearance (this also includes colored and painted coatings);
- hard (usually> 90 microns) - first of all, an increased microhardness of the surface is needed. They can also perform the function of electrical insulation);
- electrical insulating (40-90 microns) - the value of the breakdown voltage is estimated;
- thin-layer (up to 9-15 microns) - used, as a rule, for painting, or to maintain the gloss of the surface after coating;
- ematal;
- coatings with combined properties.
Figure 1 - Anodizing of metal. Examples.
An.ox.hard is a hard anodizing of aluminum, which differs from the standard An.Ox coating in its high thickness and features of the application process. In some cases, the thickness of a hard coating reaches hundreds of micrometers, while in a conventional coating it is measured in tens. The high thickness and hardness of An.Ox provides unsurpassed wear resistance of the aluminum surface.
The following are used as electrolytes:
- Low-aggressive phosphoric, citric, boric acid;
- Aggressive sulfuric, sulfosalicylic acid, chromic anhydride.
Metal anodizing always takes place at an increased voltage, most often from 12 to 120 V. Sometimes the voltage can reach enormous values for electroplating - up to 600V.
The reaction products released at the anode can:
- completely dissolve (no coating is formed);
- create on the metal surface a tightly adhered thinnest (tens of nanometers) compact electrical insulating oxide coating;
- partially dissolve in the electrolyte and form a porous oxide coating tens and hundreds of micrometers thick.
After application, the porous coating can remain "as is", seal in water, or fill. In the first case, the coating is perfect for applying paints and varnishes and pasting. In the second, the coating retains its silvery color and becomes more corrosion-resistant. In the third case, the coating can be given a color without applying paints and varnishes. More about this is written in section 6.
2. Composition and structure of aluminum oxide in the coating after coating.
Anodic oxide coatings on aluminum can be thin non-porous and thick porous.
When thin coatings are obtained in weak, low-corrosive electrolytes, an oxide is formed on the metal surface according to the reaction:
2Al + 3H2O - 6e → Al2O3 + 6H+
An illustration of the reaction is shown in Figure 2.
Figure 2 - Scheme of the formation of a thin oxide film in low-corrosive electrolytes.
All these solutions operate at a high temperature of 70 to 95 °C, which is necessary to increase the conductivity of the solution and reduce energy costs. And yet the voltage on the bath remains very significant - 150-600 V. The processing time is 15-30 minutes, and the thickness of the coatings does not exceed a fraction of a micron. Due to the low porosity, thin anodic oxide coatings are poorly colored.
Thick porous anodic oxide coatings are obtained from aggressive solutions (for example, from sulfuric acid solution). In coatings obtained from corrosive electrolytes, two layers are usually distinguished (Figure 3):
1) Thin non-porous barrier layer adjacent to the metal (1), formed from the condition of 0.008 - 0.012 μm per 1 V of applied voltage, and usually constituting 0.01 - 0.03 μm.
2) A thick porous layer (2), which is a system of conical pores penetrating the oxide film and having a thickness of several micrometers to millimeters.
Figure 3 - The structure of layers of aluminum oxide obtained from corrosive electrolytes.
The structure of the thick porous anodic oxide coating is confirmed by the results of electrochemical impedance spectroscopy (Figure 4).
Figure 4 - Bode plots for aluminum grade Al 6061, anodized in sulfuric acid electrolyte, followed by compaction in water when immersed in a 3.5% sodium chloride solution for a specified time. Left - Bode module, right - Bode phase.
The following areas can be traced in the graphs of the Bode module:
- High impedance values at frequencies ≤ 1 Hz clearly indicate the characteristics of the barrier layer of the anodic coating.
- The quasi-horizontal area in the Bode modulus plot and the corresponding minimum area in the Bode phase plot characterize the behavior of the resistance of the porous layer.
- The steep part at higher frequencies in the Bode modulus plot characterizes the capacitive behavior of the porous layer.
An equivalent electrical diagram of a porous anodic oxide coating with a seal in water is shown in Figure 5.
Figure 5 - Equivalent electrical circuit of a porous anodic oxide coating with compaction in water: Rsol - resistance of the electrolyte, Ro and Co - resistance and capacity of the outer crystal layer, Rpw and Cpw - resistance and capacity of the pore wall, Rp and Cp - resistance and capacity of the pore body, Rb and Cb are the resistance and capacitance of the barrier layer.
With regard to the composition of the anodic oxide coatings, the thin non-porous films are mainly anhydrous aluminum oxide, which in its pure form is located at the border with the metal. In coatings of this type, from 0.6 to 20% boric anhydride (electrolytes with boric acid), as well as a significant amount of other ions, are introduced. A small part of the hydrated oxide Al2O3 * H2O (boehmite) is found at the oxide-electrolyte interface.
Thick porous anodic oxide coatings consist mainly of amorphous aluminum oxide and, in part, γ-Al2O3. During the hydration of the oxide, due to the flow of electrolyte through the pores to their bottom, both physical adsorption of water and the formation of a phase of boehmite Al2O3 * H2O or bayerite Al2O3 * 3H2O can occur. The total water content in coatings obtained from sulfate electrolytes reaches 15%, while the barrier layer can contain up to 2% water. Wall hydration increases from the bottom to the mouth. Most researchers are inclined to believe that the water in the coating is not chemically bound, with the exception of the surface layers, where it is part of the boehmite.
Thick coatings also contain a significant amount of electrolyte anions - up to 20%. For example, the mass fraction of sulfates can be up to 14%. Electrolyte ions are unevenly distributed in the film: most of the sulfate ions are located in the surface layers of the oxide layer (up to 0.5 μm), throughout most of the porous layer, the content of sulfate ions is constant (about 10%), and there are no electrolyte ions in the barrier layer ... 50-60% of anions are retained by capillary forces in the pores, the rest are firmly bound to oxides and distributed fairly evenly over the thickness of the coating. The latter are called structural anions.
The metal impurities contained in aluminum alloys, for the most part, remain in the oxide film (iron, copper, silicon, magnesium, calcium). Zinc and titanium are present as traces with a content of 0.1%.
In colored oxide films, inclusions of carbon, sulfur and their oxide compounds are found, which give color.
Most of the ions are not removed from the coating by long-term rinsing with water at a high temperature, or by using other solvents. Such a high bond strength of ions with the substance of the anode film in the absence of simple stoichiometric ratios between the embedded ion and aluminum oxide indicates the incorporation of ions into the elementary formations of the film. Apparently, part of the anions is held by capillary forces in the pores of the coating, while the other part is chemically bonded to the walls of the porous layer.
With an increase in the amount of impurities in the metal, an increase in the temperature of the electrolyte and the density of the anode current, the irregularity of the microstructure of oxide coatings increases - the perpendicularity of growth of cells and pores is disturbed, and their parameters become more uneven. The most chaotic structure is observed in films formed on aluminum alloys in solutions of chromic and orthophosphoric acids.
Figure 6 - The original surface of aluminum before anodizing.
Figure 7 - Surface of aluminum with oxide, after anodizing in sulfuric acid electrolyte.
As can be seen from Figures 4 and 5, after anodizing, microroughnesses caused by mechanical processing disappear on the aluminum surface. In this case, a dense porous oxide film is formed.
If we separate the porous and barrier layers, then the following picture can be seen (Figure 8):
Figure 8 - An example of an industrial anodized aluminum surface: a - a replica of a porous layer, b - a replica of a barrier layer, c - a schematic representation.
3. Theories of the formation of aluminum oxide films during anodizing.
There are two theories of the formation and growth of anodic oxide coatings: structural-geometric and colloidal-electrochemical.
3.1 Structural-geometric theory (Keller cells).
From the standpoint of this theory, when an anode voltage is applied to the aluminum electrode (that is, connecting it to the "plus"), a compact oxide film is first formed (a barrier layer with a thickness of 1-1.1 nm / V), which has a hexagonal cellular structure, and the growing coating will be repeat it.
The outer part of the cells in aggressive electrolytes that dissolve the oxide begins to break down in defective places and turn into a porous coating. The destruction of the barrier layer, leading to the formation of a pore, occurs, in the opinion of some researchers, in the center of the cell, in the opinion of others - at the junction of the cells.
Thus, under the influence of local influences of electrolyte ions, pores arise in the barrier layer, the number of which is inversely proportional to the voltage. The pore diameter and their number depend on the nature of the electrolyte and the process mode. In the pore, the thickness of the barrier layer decreases, and, as a consequence, the electric field strength increases, while the ion current density increases together with the oxidation rate. But, since the temperature in the pore channel also increases, which facilitates the etching of the pore, dynamic equilibrium sets in, and the thickness of the barrier layer remains practically unchanged. The size of the cells increases with an increase in the forming voltage. An example of a Keller cell is shown in Figure 9. The pore shape differs from one author to another - from round to "asterisk".
Figure 9 - Keller cell.
The growth of the anode-oxide layer occurs at the bottom of the formed pores due to the transformation of ever deeper layers of metal into oxide. Subsequently, under the action of the electrolyte, the oxide forming the cell walls is hydrated. In this case, the adsorption of water, electrolyte anions and products of the anodic reaction occurs.
3.2 Colloidal-electrochemical theory of Bogoyavlensky.
The presence of electrolyte anions in the composition of the oxide layer forced scientists to associate the growth and features of its structure with the colloidal structure.
From the standpoint of the theory of Bogoyavlensky (Figure 10), the formation of anodic oxide films begins with the appearance of monons - the smallest oxide particles with adsorbed electrolyte anions. The nucleation of monons occurs as a result of the meeting of ion flows. Monones are the embryos of future micelles.
With an increase in the number of monons, they turn into polyions - fibrous rod-shaped micelles of colloidal degree of dispersion, which form the skeleton of an oriented aluminum oxide gel. Anions of the electrolyte are incorporated into it, partially losing their hydration shell.
The adsorption of anions and water, carried out through intermicellar pores, causes a negative charge of monon and micelles, forcing them to adhere tightly to the anode and coalesce with the metal, preventing the fusion of micelles into a porous layer. When viewed in this way, the pores represent a natural intermicellar space.
Along with the formation of micellar layers with the participation of anions, coupled processes of dissolution of the formed oxide occur.
Figure 10 - Illustration of the theory of Bogoyavlensky.
It is interesting to note that the sizes of the Keller cells are close to those of the micelles of the Al (OH)3 gel. The interpretation of the growth mechanism of the anode film from the standpoint of colloidal chemistry makes it possible to explain the introduction of anions and cations of the electrolyte and individual components of the oxidized alloy into its structure. In this case, the conjugation of the processes of oxide formation and its dissolution in the electrolyte is also taken into account by the colloidal theory.
3.3 Modern studies of the structure of the coating.
Now it should be noted that the structure of anodized aluminum, in fact, can be very far from the ideal, described in theory. In particular, the theory speaks of regular hexagonal cells, in the center of which there is one pore. In fact, such a structure can only be obtained by special methods, for example, by multistage anodizing in certain modes. Examples of such “correct” coatings are shown in Figure 11. A deeper description of nanostructured anodic oxide coatings is beyond the scope of this article.
Figure 11 - Examples of ideal and near-ideal cells of a porous layer in an anodic oxide coating on aluminum.
More often, you can observe more "dirty" options. Examples of them were shown at the beginning of the article.
In addition, theories do not imply the possibility of pore branching, which is actually observed.
4. Features of the growth of aluminum oxide during anodizing.
The formation of the oxide layer occurs at the bottom of the pores, where only a thin barrier layer, the thickness of which practically does not change during processing, serves as an obstacle to the passage of electric current. From this point of view, it is possible to increase the thickness of the oxide layer without significantly increasing the voltage on the bath. The resulting pores are in the form of a cone, expanding towards the outside of the coating, since this part is exposed to the aggressive action of the electrolyte for a longer time.
It should be noted that the formation of a porous structure is a necessary condition for the growth of the oxide layer. Aluminum oxide is a poor conductor of electricity, and the pores, although filled with electrolyte, have a very small diameter, so the resistance of the anode is many times higher than the resistance at the cathode and the resistance of the electrolyte. The change in the potentials of the electrodes themselves due to polarization is insignificant in comparison with the applied voltage; therefore, the change in voltage over time at a constant current density is determined by the change in the ohmic resistance of the anode.
If the process is carried out at a constant current density, i.e. at a constant rate of oxide formation, the growth of the film will be inhibited by the increasing resistance of the electrolyte in the pores. Further growth requires either an increase in the applied stress or pore etching. In practice, the second factor prevails. This is facilitated by the significant release of heat in the process of anodic oxidation, and the bulk of the heat is released in the barrier layer at the bottom of the pores. It is believed that during anodic oxidation in 15% sulfuric acid at 21 °C and a current density of 1.29 A / dm2, the conditions at the base of the pores correspond to 53% sulfuric acid solution at a temperature close to boiling (about 128 °C). In this case, the anode temperature rises by 10-20°C, depending on the conditions of the process. Therefore, the growth of the oxide film at a constant current density is accompanied by a continuous increase in the rate of oxide dissolution. The limiting film thickness is reached when the rate of its formation under the action of an electric current becomes equal to the rate of chemical dissolution by the electrolyte. Excessive overheating of the electrolyte at the base of the pores and a local increase in its aggressiveness can lead to etching of the oxide layer and the production of poor-quality coatings with increased porosity and poor adhesion to the metal.
The rate of dissolution of the oxide film in sulfuric acid increases with increasing temperature, while the activation energy for dissolution of the anode film is estimated at 17 kcal/mol, which indicates the control of the rate by the kinetic stages of the chemical dissolution process. The rate of chemical dissolution of aluminum oxide is relatively high, especially in aggressive sulfuric acid solutions.
Enhanced dissolution of oxide stimulates an increase in the concentration of aggressive acid, temperature and duration of the process:
- at 20 ° C in a 20% sulfuric acid solution for commercially pure aluminum, alloys D16 and AL9, the dissolution rate is 0.2, 0.14 and 0.18 g/dm2∙h.
- when the temperature of the electrolyte is doubled, the dissolution rate increases 6 - 7 times.
- with an increase in the concentration of sulfuric acid from 180 to 350 g/l, the dissolution rate increases by about 15%.
The dissolution of oxide is expressed not only in etching the surface layer of the forming coating, but also in an increase in its porosity. The presence of copper and magnesium in aluminum alloys also slightly increases the rate of dissolution of oxide in sulfuric acid.
Thus, the ratio of the rates of oxide formation and its chemical dissolution predetermines both the thickness and structure of the obtained anodic oxide coatings on aluminum.
Due to the fact that the formed oxide layer has a high resistance, the electric current during oxidation is automatically redistributed to those areas where the resistance is lower. Thus, conditions are created for obtaining an oxide layer uniform in thickness on parts of complex configuration. Therefore, the scattering power of electrolytes for anodic oxidation of aluminum and its alloys is very high. However, it should be borne in mind that with insufficient heat removal from the forming coating, the possibility of local etching of individual sections of the coating arises, which will not be compensated by an increase in the current density in these sections. This will lead to local defects in the coating, up to and including its complete absence. Gradually unsatisfactory conditions for the formation of the coating can cover the entire part.
Due to the partial dissolution of the base metal during anodic oxidation, the current efficiency is always less than 100%. It decreases with increasing temperature and duration of electrolysis. For example, when the D16 alloy is oxidized in sulfuric acid at a temperature of 7 °C, the current efficiency is practically independent of time and is 85%, but if the process is carried out at 20 °C, then the current efficiency drops from 50-60% during the first 20 min up to 15-30% with oxidation for 90 min. Electricity consumption for gas evolution is small and at moderate current densities (up to 1-2 A / dm2) does not exceed several percent, but it can increase with an increase in the current density and the amount of alloying elements in the alloy being processed.
5. Properties of oxide coatings on anodized aluminum.
5.1 Corrosion resistance and porosity.
Anodic oxide coating on the surface of aluminum and its alloys has a beneficial effect on its corrosion resistance in many environments where the oxide is more resistant than the base metal. They successfully protect aluminum from atmospheric corrosion, in neutral and slightly acidic solutions of inorganic salts:
- the durability of anodic oxide coatings in the sea atmosphere and sea water is confirmed by long-term operation of oxidized aluminum parts.
- anodic oxidation reduces aluminum corrosion in acetylene, sulfur dioxide, boric acid and benzenesulfonic acid, ethanol and ethanol solutions.
- in the presence of moisture, the coating hydrates along the pore walls with the formation of boehmite or hydrargilite, which contributes to an increase in the weight of the coating, its compaction and a decrease in the corrosion rate over time.
- in chloride-containing media, the corrosion process has a pronounced local character, flowing through the pores of the coating; it is accompanied by the formation of aluminum hydroxochlorides of variable composition, gradually turning into hydroxide, which also contributes to the gradual clogging of pores and slowing down corrosion.
Figure 12 shows the corrosion curves for pure aluminum and aluminum with anodic oxide coatings.
Figure 12 - Corrosion curves for pure and anodized aluminum: SAA - water sealed coating, IC - inorganic dye filled coating, BD - organic dye filled coating, EC - electrochemical staining, Bare Al - pure aluminum. Corrosive environment - 3.5% sodium chloride solution.
For pure aluminum, the corrosion resistance is 0.5953 kΩ, the corrosion current is 130.86 mA. After anodizing, the corrosion resistance rises to 24.216 kΩ and the corrosion current drops to 7.494 mA.
According to the corrosion curves, it can be seen that the corrosion potential shifts to the negative region in the series SSA, IC, BD, EC, pure Al. In the same series, the corrosion resistance of aluminum also decreases.
The atmospheric corrosion rate of pure aluminum is 0.4284 mm / year. After anodizing, the corrosion rate decreases to 0.0817 mm / year.
Microimages of the surface of anodized aluminum with different types of sealing and filling before and after corrosion are shown in Figure 13.
Figure 13 - Microimages in the topographic contrast mode of anodic oxide coatings: SAA - metal anodizing with compaction in water; BD - filled with black organic dye; IC - filled with inorganic dye; EC - with electrochemical coloration in tin salts.
Based on practice, the minimum thickness of oxide coatings that provide protection for products is selected according to the operating conditions:
- in closed rooms with artificially controlled climatic conditions - 9 microns;
- outdoors in rural, forest, mountainous areas far from industrial facilities - 15 microns;
- outdoors in urban and coastal atmosphere - 21 microns;
- outdoors in the industrial atmosphere of the northern coast (chlorides not less than 10 mg/m2 per day) with prolonged moisture - 24 microns;
- when applying varnishes and paints, it is allowed to reduce the thickness of the coating to 9 microns when operating in the open air and to 15 microns in a marine atmosphere.
The best corrosion resistance was found for coatings made on pure aluminum.
The addition of copper, silicon, iron, magnesium, and manganese to aluminum improves the mechanical properties of the alloy, but impairs the protective ability of the resulting oxide coatings. Silicon and intermetallic compound Al6Mg oxidize much more slowly than aluminum and remain in the form of inclusions in the coating. On the contrary, intermetallic compounds Al3Mg2, Al2Cu, CuAl2, CrAl7, Co2Al9, Co2Al5, Co4Al13, Al7CuFe, Al6CuNi are easily destroyed and increase the porosity of the coating. Thus, the corrosion resistance of coatings with a thickness of 2.5-10 microns, obtained on the AD1 alloy, is 6-7 times higher than that of coatings on the 1915 and AD31 alloys, and 2-3 times higher than that of the coatings on the AMg2AP alloy. Increasing the thickness of the coatings to 15 µm smoothes these differences.
The corrosion resistance of oxide coatings increases with an increase in the thickness of the barrier layer, which accounts for approximately 1/3 of the corrosion resistance provided. At the same time, an increase in the thickness of the porous part of the coating has a beneficial effect on their corrosion resistance only in the case of relatively thin coatings, while a further increase in thickness is accompanied by an increase in the pore diameter and a decrease in protective properties.
The porosity of the coatings obtained under various conditions is shown in the table below:
Electrolyte |
Working temperature |
Bath voltage |
Number of pores per 1 m2 n*1012 |
Sulfuric acid (15%) |
10 |
15 20 30 |
79,1 53,1 28,4 |
Chromic acid (3%) |
29 |
20 40 60 |
22,2 8,28 4,29 |
The porosity of the oxide surface layer varies from 15 to 40% depending on the alloy grade and anodizing mode. With an increase in the temperature of the electrolyte, the porosity increases.
The corrosion resistance of the films slightly increases with an increase in their thickness, however, the accompanying increase in coating porosity and the formation of cracks in the surface layer sharply increases the rate of corrosion damage.
The protective ability of anodic oxide coatings can be significantly improved by filling the pores in various solutions containing substances that inhibit corrosion. A promising method for increasing the corrosion resistance of anodic-oxide coatings should be considered the creation of combined coatings in which the porous oxide plays the role of an adsorption layer that holds the organic polymer material resistant to aggressive media.
5.2 Electrophysical properties of aluminum oxide.
Protective and decorative oxide coatings obtained in aqueous solutions of sulfuric acid have high electrophysical characteristics. The microhardness of the oxides obtained at a current density of 0.5-2 A / dm2 is H 300 - 500, while that of commercially pure aluminum is about H 30. The microhardness of anode films, measured on a PMT-3 microhardness tester, on industrial aluminum can reach H 600 , and on chemically pure aluminum - H 1500. In this case, the microhardness of the obtained coatings is uneven in thickness: the layers adjacent to the metal have a microhardness 50-100% higher than the outer ones, which is associated with the higher porosity of the surface layers.
The microhardness of functional anodic oxide coatings depends on the nature of the aluminum alloy and is (GPa):
- on pure aluminum - 4.9-5.1,
- on alloy AB - 4.7-4.9,
- on AL type alloys - 4.4-4.7,
- on alloy D16 - 3.24-3.53.
The highest quality coatings are formed on pure aluminum and its alloys with magnesium, the least quality - on alloys with a copper content of more than 4.5% (D1, D16, D20).
Anodic oxide is a good dielectric: the specific resistance is on average 4 ∙ 1015 Ohm ∙ cm, the breakdown voltage can reach 1 kV or more.
To improve the strength and electrical insulating properties, thickened coatings are obtained (usually 40 - 90 microns, although oxidation to a thickness of several tenths of a millimeter is possible). In some industries (instrumentation, mechanical engineering, aviation technology), the thickness of the coatings is limited to 75 microns due to the possibility of cracking on thick coatings and defective coatings on parts with sharp edges, which sharply reduces the electrical insulating capacity and wear resistance.
The electrophysical properties are affected not only by the thickness and porosity, but also by the structure of the coating; therefore, the results are highly dependent on the composition of the electrolyte and the processing mode.
Thus, coatings obtained in a sulfate solution with modifying additives on AD0 aluminum with a thickness of 84 μm and a porosity of 14% had a breakdown voltage of 2.5 kV, while coatings with a thickness of 165 μm with the same porosity had a breakdown voltage of only 1.5 kV.
Films with a thickness of 161 μm with a porosity of 9% showed a breakdown voltage of 1.83 kV, and coatings with a thickness of 154 μm with a porosity of 23% showed a breakdown voltage of 2.33 kV.
The breakdown stress on cast alloys is lower than on wrought alloys.
The thermal insulation properties of oxidized aluminum alloys are higher compared to non-oxidized metals. The thermal conductivity of aluminum oxide is 0.004-0.012 J / (cm ∙ s ∙ °С), which is 200-500 times lower than that of pure aluminum. The thermal emissivity of anodized surface is 10 times higher than that of pure metal.
Thick films on aluminum alloys are highly resistant to high temperatures, withstanding heating up to 2000 ° C. Therefore, oxidation is used in the manufacture of molds for casting aluminum and magnesium alloys. With prolonged repeated exposure to high temperatures, microcracks are formed on the oxidized surface, due to the difference in the values of the linear expansion coefficient of the anode film and aluminum.
The growth of the oxide layer is associated with the partial dissolution of the base metal and the surface layer of the formed oxide, which affects the change in the size of the part during processing. In the first half hour of processing, the size of the oxidized part increases by 1 - 2 microns, but then the size decreases to -2 microns after 1 hour of processing and further. Therefore, when applying thick coatings, it is necessary to take into account the change in the size of the parts during the coating process. The increase in the size of the part during the anodic oxidation of aluminum and its alloys is less than the thickness of the resulting coating. Typically, the increase in the size of the part is from 30 to 60% of the obtained thickness of the oxide layer in various electrolytes (on average 50%).
6. Sealing and painting of anodic oxide films on aluminum.
The significant porosity of the oxide coating leads to the fact that it easily adsorbs moisture, various solutions and organic substances. Porosity during anodic oxidation plays a positive role as a necessary factor for increasing the thickness of the oxide layer; however, during operation, unclosed pores are a weak spot of the coating, through which the corrosion process will proceed in the first place. Therefore, after the formation of a porous oxide, it is necessarily subjected to additional processing designed to close the pores - either with hydrated aluminum oxide (when compacted with water, in inorganic and organic substances), or various varnishes, oils and other substances with appropriate impregnation.
The ability to adsorb organic substances underlies the process of coloring anodic oxide coatings (Figure 14, 15).
Figure 14 - Examples of anodized aluminum parts filled with black dye.
Transparent and translucent protective and decorative coatings of aluminum and its alloys are painted in aqueous direct acidic organic dyes. The color of the films obtained in different anodizing electrolytes differs due to the difference in the structure, porosity, and natural color of the coatings. To obtain the required colors, a mixture of aniline dyes is used. In addition to organic dyes, inorganic ones are also used. Thus, a limited color gamut, but a greater lightfastness of anodic oxide coatings is obtained by the double exchange reaction in solutions of inorganic salts.
Figure 15 - Examples of anodized aluminum parts filled with turquoise, violet and red dyes.
Corrosion resistance of aluminum and its alloys (especially in water and aqueous media) can be significantly increased by compaction in a solution of chromium salts. Sodium salt is commonly used for economic reasons. Compositions for compacting the anodic oxide coating in bichromates are regulated by the DEF151 specification and are based on work originally carried out in the USSR and the USA.
There are compositions based on sodium dichromate with sodium carbonate or hydroxide and based on sodium dichromate. The treatment in the first anodized aluminum sealing solution takes 5-10 minutes. This time is not enough for complete compaction of the oxide anode film by hydration, but it ensures the absorption of a significant amount of chromates. The anode coating is then painted yellow. The intensity of the yellow color increases with the thickness of the coating.
The second composition for compacting anodized aluminum in dichromate without other additives implies processing over a period of time that was spent on the anodizing itself. This formulation provides a satisfactory degree of hydration, but not necessarily complete compaction.
7. Colored anodic oxide coatings.
Coatings can be colored not only by filling in dyes of organic and inorganic nature. They can also be colored directly from certain electrolytes.
If in these electrolytes aluminum and its alloys are anodized first with alternating and then direct current, then the coatings are colored from light straw to golden and bronze.
Another method of coloring anodized aluminum is electrochemical treatment in tin or nickel salts.
Хотите стать нашим клиентом?
Просто оставьте Вашу заявку, заполнив форму справа и мы свяжемся с Вами в ближайшее время. Спасибо!
Отправляя заявку, Вы даете согласие на обработку Ваших персональных данных. Ваши данные под защитой.